|
Monophyly - Paraphyly - Polyphyly
An introduction
|
|
The figure below shows a collection of
objects of different shapes and colors.
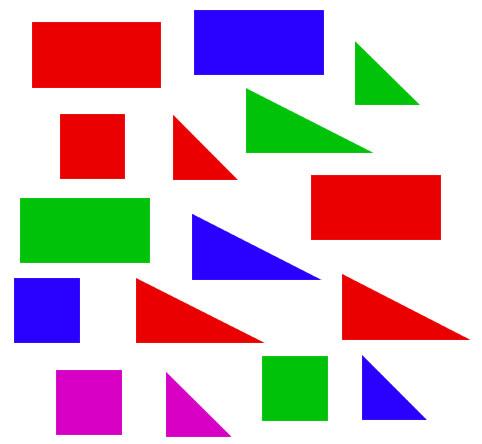
We
can make groups of objects according to criteria that we choose and
then give names to the groups. For example, we can group together all
objects with the same shape:
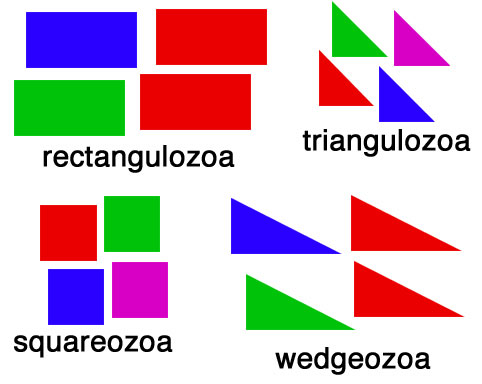
Choosing the shape as the criterion for group formation leads to four
groups of objects.
We could also choose the color as the criterion to form groups from the
initial set of objects:
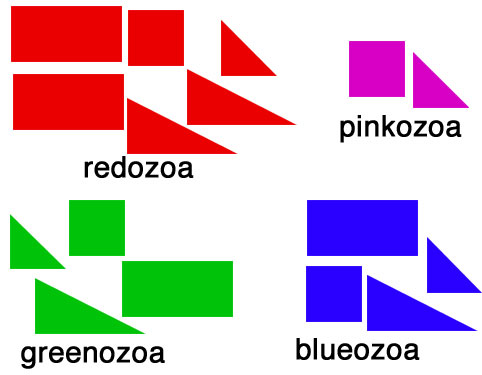
This criterion also leads to four groups of objects, but these are
different from the groups formed before.
We have now two different systems for grouping the objects. The
criterion
for system 1 is the shape of the objects, and the criterion for system
2 is
the color of the objects. Neither system is better or worse than the
other, and none is "the correct" system, since the criteria (shape,
color) were chosen arbitrarily. Systems like these are called
"artificial" or "non-natural".
However, in some cases there can be a preexisting system that links the
objects. This system is not man-made and is therefore called "natural".
The objects might be linked, because the descended from each
other:
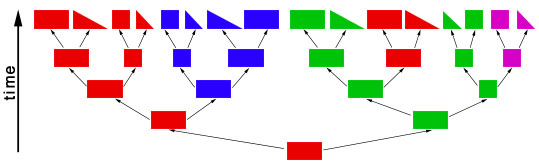
In this scenario the objects have evolved in several steps from a red
rectangle. In the first generation, the red rectangle produced two
offspring: one of them retained the original shape and color, but the
second offspring evolved a new color, green. Evolutionary changes of
shape and color also occurred in the
next generations, leading to the set of objects that we already know
from our previous considerations. The objects are now linked by descent
and, of course, we now want to make groups that join together related
objects, in order to obtain "groups of relatives", "families" or
"natural groups".
Two groups in this tree already appeared in our previous systems: all
pink objects derive from a common ancestor and all blue objects derive
from a common ancestor:
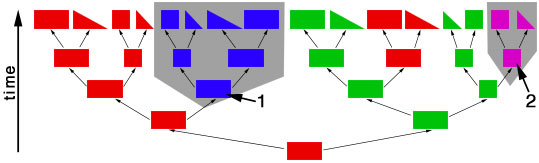
Arrow "1" points to the common ancestor of all blue objects
(blueozoa) and arrow "2" points to the common ancestor of all pink
objects (pinkozoa). The groups blueozoa and pinkozoa contain all descendants of the common
ancestor. A group like this is called monophyletic,
because they descend from a single
ancestor, and it is called holophyletic, because it contains all descendants of this ancestor.
These two groups are "groups of relatives" and thus "natural groups".
But what about the other groups from our previous systems? Let us first
consider the greenozoa:
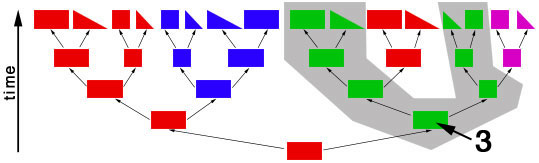
All greenozoa also derive from a single common ancestor (arrow "3").
The
greenozoa are
thus monophyletic. However, the greenozoa do not contain all
descendants of this ancestor, because some of the red and all pink
objects also derive from this ancestor. The greenozoa are thus not holophyletic. Groups that are
monophyletic, but not holophyletic, are called paraphyletic.
Let us now consider the redozoa:
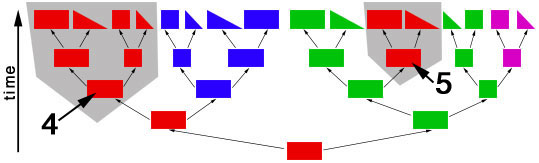
Originally we grouped these objects together, because they all share
the character "red color". Now we see that not all red objects are
directly related, because they derive from different ancestors (arrow
"4" and arrow "5"). Thus, the redozoa is a group that contains
unrelated objects characterized by a character that is identical in all
objects, but that has evolved more than once. A group like this is
called polyphyletic.
All groups that we have formed by using the shape of the objects also
are polyphyletic. The example below shows the distribution of the
rectangulozoa in the tree:
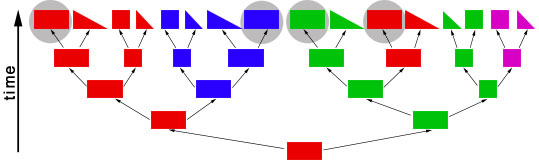
In summary, there are three different kinds of groups that we can form
in the evolutionary tree:
1) monophyletic holophyletic groups (mostly simply called monophyletic
groups)
2) monophyletic paraphyletic groups (mostly simply called paraphyletic
groups)
3) polyphyletic groups
All objects in the evolutionary tree are linked by descent. Only
monophyletic holophyletic groups reflect this fact. Thus, for a natural
system only monophyletic holophyletic
groups are useful. We have seen that we obtained the desired
monophyletic holophyletic groups only in some cases, depending on the
character that we used. Thus, there are "good" and "bad" characters.
A "good" character is e.g. "blue color", because it evolved only once
in the ancestor of the blue group and is retained in all descendants.
The blue color is thus an evolutionary innovation that occured in the
ancestral blue object and still characterizes all extant objects of
this ancestor. Such characters are called apomorphic characters or apomorphies. The apomorphy "blue
color" characterizes the entire group
(blueozoa). The scientific term for this is: the blue color is the autapomorphy for the blueozoa. But
the blue color also characterizes each
and every member of the blueozoa. The scientific term for this
is: the blue color is the synapomorphy
for the blue rectangle, blue square, blue triangle and the blue wedge.
A character somewhat between "good" and "bad" is e.g. "rectangle
shape". There are several objects with this character and these objects
are not all directly related. Thus, this character leads to the
polyphyletic group "rectangulozoa". Nevertheless the shape of these
objects ultimately derives from a common ancestor that is also the
ancestor of other objects: the rectangular shape is the ancestral
state. Such a character is called plesiomorphic character or plesiomorphy. Plesiomorphies are
normally useless for making groups. However, if a monophyletic group is
already known based on other apomorphic characters, then plesiomorphies
can nevertheless be useful to determine the direction of evolution of
characters.
Some characters evolve too quickly and can change back
and forth frequently. In the example, shape is a character of this
kind. These characters do not carry a phylogenetic
signal and are useless
for making groups, because these groups will be polyphyletic.
Another character kind are characters that evolved in parallel in
different branches of the tree. In the example, the red color is a
character of this kind. The evolution of similar characters in
different branches is called convergent
evolution. The resulting similarities are called homoplasies. These characters also
are useless for making groups, because these groups will also be
polyphyletic.
In practice, of course, apomorphies, plesiomorphies, and homoplasies
can be difficult to identify. These problems are the cause for the many
different hypotheses about metazoan phylogeny.
A real example for a paraphyletic group is the Reptilia (reptiles).
This group is based on characters like skin scales or the morphology of
the heart, that once were believed to be apomorphic, but now are
regarded as plesiomorphic characters. The ancestor of the Reptilia is
also the ancestor of the birds (Aves). Thus the group Reptilia is
monophyletic, but not holophyletic.
A real example for a polyphyletic group is the Vermes (worms). This
group is based mainly on the long thin shape of the body. This shape
has evolved several times independently and is thus a homoplasy.
|
This page has been
updated on August 20, 2008
This site is online since May 31, 2005
Copyright © by Nikola-Michael Prpic. All rights reserved.
|
|
|